Physical and physicochemical methods
according to Pharmacopoeias (PH.EUR. / USP / JP).
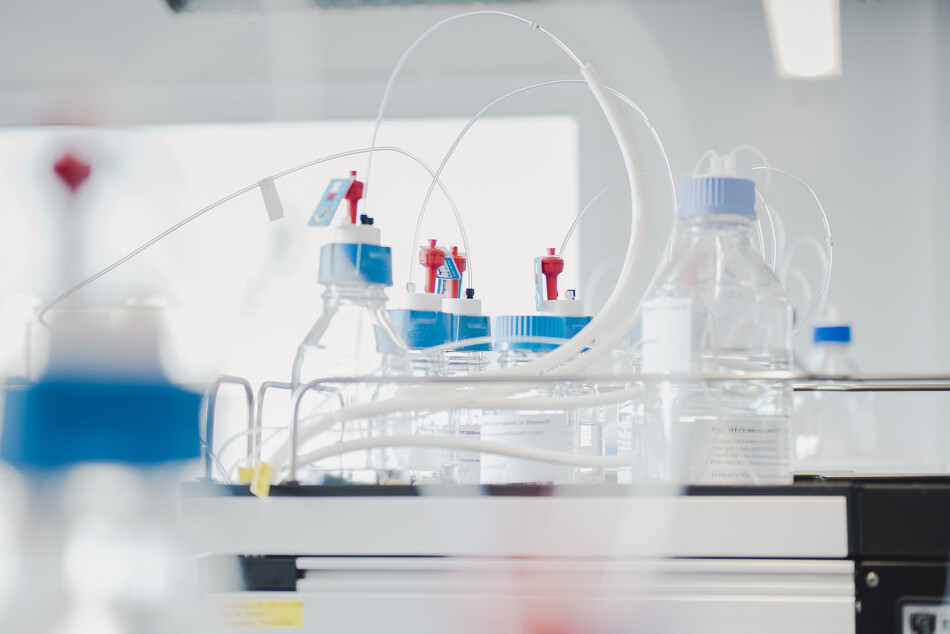
Overview of methods:
Opalescence is the effect of light being absorbed or scattered by submicroscopic particles or optical density inhomogeneities. The absence of any particles or inhomogeneities in a solution results in a clear solution.
A solution is colourless if it has the appearance of water R or the solvent used for the preparation of the solution to be examined, or is not more intensely coloured than reference solution B9.
The pH of an aqueous solution is defined as the negative logarithm of the activity of its hydrogen ions, expressed conventionally as the hydrogen ion concentration of the solution. For practical purposes, its definition is an experimental one. The pH of a solution to be examined is related to that of a reference solution (pHs) by the following equation
Determine the approximate pH using a pH indicator strip R. Alternatively, pH indicators such as those described in Table 2.2.4.-1 can be used.
The relative density Equation of a substance is the ratio of the mass of a certain volume of a substance at temperature t1 to the mass of an equal volume of water at temperature t2.
The refractive index of a medium with reference to air is equal to the ratio of the sine of the angle of incidence of a beam of light in air to the sine of the angle of refraction of the refracted beam in the given medium.
Optical rotation (also known as optical activity) is the property displayed by chiral substances of rotating the plane of polarisation of linearly polarised light.
The dynamic viscosity or viscosity coefficient η is the tangential force per unit surface, known as shearing stress τ and expressed in pascals, necessary to move, parallel to the sliding plane, a layer of liquid of 1 square metre at a rate (v) of 1 metre per second relative to a parallel layer at a distance (x) of 1 metre.
The determination of viscosity is carried out using a suspended-level (Ubbelohde-type) capillary viscometer of appropriate size at a temperature of 20.0 ± 0.1 °C, unless otherwise prescribed. The time required for the level of the liquid to drop from one mark to the other is measured.
The principle of the method is to measure the force acting on a rotor (torque) when it rotates at a constant angular velocity (rotational speed) in a liquid. Rotating viscometers are used for measuring the viscosity of Newtonian (shear-independent viscosity) or non-Newtonian liquids (shear dependent viscosity or apparent viscosity).
The distillation range is the temperature interval, corrected for a pressure of 101.3 kPa, within which a liquid or a specified fraction of a liquid, distils under the following conditions.
The boiling point is the corrected temperature at which the vapour pressure of a liquid is equal to 101.3 kPa.
The melting point determined by the capillary method is the temperature at which the last solid particle of a compact column of a substance in a tube passes into the liquid phase (i.e. clear point).
For certain substances, the following method is used to determine the melting point (also referred to as slip point and rising melting point when determined by this method).
In an amperometric titration, the end-point is determined by following the variation of the current measured between 2 electrodes (either one indicator electrode and one reference electrode or 2 indicator electrodes) immersed in the solution to be examined and maintained at a constant potential difference as a function of the quantity of titrant added.
In a potentiometric titration (volumetric titration with potentiometric end-point determination) the end-point is determined by recording the variation of the potential difference between 2 electrodes (either 1 indicator electrode and 1 reference electrode, or a combined electrode) immersed in the solution to be examined as a function of the volume of titrant added.
Fluorimetry is a procedure which uses the measurement of the intensity of the fluorescent light emitted by the substance to be examined in relation to that emitted by a given standard.
Atomic emission is a process that occurs when electromagnetic radiation is emitted by excited atoms or ions. In atomic emission spectrometry the sample is subjected to temperatures high enough to cause not only dissociation into atoms, but also to cause significant amounts of collisional excitation and ionisation of the sample atoms to take place. Once the atoms and ions are in the excited states, they can decay to lower states through thermal or radiative (emission) energy transitions and electromagnetic radiation is emitted. An emission spectrum of an element contains several more lines than the corresponding absorption spectrum.
Atomic absorption is a process that occurs when a ground state-atom absorbs electromagnetic radiation of a specific wavelength and is elevated to an excited state. The atoms in the ground state absorb energy at their resonant frequency and the electromagnetic radiation is attenuated due to resonance absorption. The energy absorption is virtually a direct function of the number of atoms present.
This chapter provides general information and defines the procedures used in element determinations by atomic absorption spectrometry, either atomisation by flame, by electrothermal vaporisation in a graphite furnace, by hydride generation or by cold vapour technique for mercury.
Atomic absorption spectrometry is a technique for determining the concentration of an element in a sample by measuring the absorption of electromagnetic radiation by the atomic vapour of the element generated from the sample. The determination is carried out at the wavelength of one of the absorption (resonance) lines of the element concerned. The amount of radiation absorbed is, according to the Lambert-Beer law, proportional to the element concentration.
Infrared absorption spectrophotometry (also known as infrared (IR) spectroscopy) is based on the interaction of infrared radiation with matter. As a result of interaction between a molecule and IR radiation, absorption of frequencies specific to that molecule can occur, and some intermolecular and intramolecular vibrations can be excited to higher vibrational levels. This results in an infrared absorption spectrum with characteristic bands that correspond to the functional groups of the molecule.
Ultraviolet and visible (UV-Vis) spectroscopy (or spectrophotometry) is based on the ability of atoms, molecules and ions to absorb light at specific wavelengths in the ultraviolet (approximately 180-400 nm) and visible (approximately 400-800 nm) range. This absorption is associated with changes in electronic energy in the form of temporary transitions of electrons to an excited state at a higher energy orbital. As each energy level of a molecule or molecular ion also has associated vibrational and rotational sub-levels, this results in many permitted transitions, which are generally impossible to separate, thereby producing absorption bands rather than sharp lines. These bands are characteristic of the functional groups and bonds in a molecule.
Thin-layer chromatography is a separation technique in which a stationary phase consisting of an appropriate material is spread in a uniform thin layer on a support (plate) of glass, metal or plastic. Solutions of analytes are deposited on the plate prior to development. The separation is based on adsorption, partition, ion-exchange or combinations of these mechanisms and is carried out by migration (development) of solutes (solutions of analytes) in a solvent or a suitable mixture of solvents (mobile phase) through the thin layer (stationary phase).
Gas chromatography (GC) is a chromatographic separation technique based on the difference in the distribution of species between two non-miscible phases in which the mobile phase is a carrier gas moving through or passing the stationary phase contained in a column. It is applicable to substances or their derivatives that are volatilised under the temperatures employed.
Liquid chromatography (LC) is a chromatographic separation technique based on the difference in the distribution of species between 2 non-miscible phases, in which the mobile phase is a liquid that percolates through a stationary phase contained in a column.
LC is mainly based on mechanisms of adsorption, mass distribution, ion exchange, size exclusion or stereochemical interaction.
Loss on drying is the loss of mass after drying under specified conditions, calculated as a percentage (m/m).
Drying to constant mass means that 2 consecutive weighings do not differ by more than 0.5 mg, the 2nd weighing following an additional period of at least 30 min of drying under the conditions prescribed for the substance to be examined.
The potentiometric determination of the ion concentration is carried out by measuring the potential difference between two suitable electrodes immersed in the solution to be examined; the indicator electrode is selective for the ion to be determined and the other is a reference electrode.
This general chapter provides information on how to carry out electrical conductivity measurements (hereafter referred to as ‘conductivity’) of fluids, including pure liquids. It is intended for fluid applications when conductivity is used to measure, monitor or control chemical dispensing (e.g. chemical purity or ionic concentration), and other applications where the ionic character of the fluid needs to be known or controlled.
Mass spectrometry is based on the direct measurement of the ratio of the mass to the number of positive or negative elementary charges of ions (m/z) in the gas phase obtained from the substance to be analysed. This ratio is expressed in atomic mass units (1 a.m.u. = one twelfth the mass of 12C) or in daltons (1 Da = the mass of the hydrogen atom).
The ions, produced in the ion source of the apparatus, are accelerated and then separated by the analyser before reaching the detector. All of these operations take place in a chamber where a pumping system maintains a vacuum of 10− 3 to 10− 6 Pa.
The resulting spectrum shows the relative abundance of the various ionic species present as a function of m/z. The signal corresponding to an ion will be represented by several peaks corresponding to the statistical distribution of the various isotopes of that ion. This pattern is called the isotopic profile and (at least for small molecules) the peak representing the most abundant isotopes for each atom is called the monoisotopic peak.
Information obtained in mass spectrometry is essentially qualitative (determination of the molecular mass, information on the structure from the fragments observed) or quantitative (using internal or external standards) with limits of detection ranging from the picomole to the femtomole.
Total organic carbon (TOC) determination is an indirect measure of organic substances present in water for pharmaceutical use. TOC determination can also be used to monitor the performance of various operations in the preparation of medicines.
The system suitability and acceptance criteria in monographs have been set using parameters as defined below. With some equipment, certain parameters such as signal-to-noise ratio and resolution can be calculated using software provided by the manufacturer. It is the responsibility of the user to ensure that the calculation methods used in the software are equivalent to the requirements of the European Pharmacopoeia and to make any necessary corrections if this is not the case.
Inductively coupled plasma-atomic emission spectrometry (ICP-AES) is an atomic emission spectrometry method that uses an inductively coupled plasma (ICP) as the excitation source.
Inductively coupled plasma-mass spectrometry (ICP-MS) is a mass spectrometry method that uses an inductively coupled plasma (ICP) as the ionisation source. The basic principles of ICP formation are described in chapter 2.2.57 on inductively coupled plasma-atomic emission spectrometry (ICP-AES).
Within the context of the European Pharmacopoeia, the term ‘radioactivity’ is used both to describe the phenomenon of radioactive decay and to express the physical quantity of this phenomenon. In the monographs on radiopharmaceutical preparations, the detection and measurement of radioactivity are performed for different purposes: verification of the characters, identification, determination of radionuclidic and radiochemical purity, as well as determination of the radioactivity in a substance (assay).
Under these assumptions, the measurement can be qualitative, quantitative or both, depending whether it is directed to the identification of the radionuclide or the determination of its activity (rate of decay) or both of them.
Radioactive sources can produce various types of emissions, such as alpha particles, electrons, positrons, gamma- and X-rays, according to the radionuclidic composition.
Each radionuclide yields characteristic emissions, with specific energies and relative intensities. Such radiations can be detected as a result of their ionising properties in an ionisation chamber but without further characterisation; when they are detected and analysed using a spectrometer, an energy spectrum is obtained. A detailed spectrum analysis is typically used to identify radionuclides present in a sample. Spectrometry can also be used for quantitative determination of the radioactivity in sources made of a single radionuclide or radionuclide mixtures or of the individual radionuclides present.
A measurement of radioactivity is generally performed by counting the number of detected decay events (emissions). Therefore, the geometry of the sample during the measurement of radioactivity and the acquisition time strongly influence the result. In general, the measurement geometry must correspond to a calibrated geometry and the acquisition time must be long enough to reach sufficient counting statistics.
A measurement of radioactivity can be done in a stand-alone mode (e.g. using an ionisation chamber or a spectrometer) or in combination with a separation technique (e.g. radiochromatography) to account for relative contributions from different radioactive chemical species that may be present in a mixture.